Soil salinity, the accumulation of soluble salts in the soil, is emerging as a critical global issue with detrimental consequences for agricultural productivity. The primary contributors to this problem include poor irrigation practices, climate change-induced sea-level rise, and the overuse of fertilizers. As saline water infiltrates the soil, it disrupts the delicate balance of nutrients and impedes water uptake by plant roots, leading to reduced crop yields. According to a report by the Food and Agriculture Organization (FAO), soil salinization takes up to 1.5 million ha of farmland per year out of production, impacting the livelihoods of millions of farmers. The severity of this issue is underscored by projections indicating a potential 50% increase in salinized land by 2050 if current trends persist [1,2]. The problem is most predominant in arid and semi-arid regions around the world where precipitation rate is low and evapotranspiration rate is high, leading to water stress conditions and mineral leaching from the plant root zone [3].
Microorganisms in the rhizosphere or those colonizing plant tissues (endophytes) have been shown to play a crucial role in mitigating salt stress in plants, leading to enhanced crop productivity [6,11-20]. These bacteria and fungi exhibit various plant growth-promoting traits that contribute directly and indirectly to alleviating the impacts of abiotic stresses. Plant growth promoting bacteria (PGPB) directly enhance plant nutrient uptake by producing siderophores for iron sequestration, solubilizing phosphorus, and fixing nitrogen [6,11]. Additionally, they influence plant growth by providing phytohormones like indole acetic acid (IAA) or reducing ethylene production through ACC deaminase activity [6, 12-17]. Indirectly, PGPR promote plant growth by preventing or limiting damage caused by pathogenic agents such as bacteria, fungi, and nematodes [18-20]. Overall, these microorganisms offer a multifaceted approach to improving crop yields under salt stress conditions.
Our team at the University of Jordan currently focus on discovering the link between the microbiota and its metabolites on the plant response to drought and salinity stress. Identifying key transcriptional factors involved in plant growth, development and abiotic stress response.
Key results and findings
In collaboration with colleagues at the faculty of agriculture and at Hamdi mango research centre for scientific research at the University of Jordan. We have successfully isolated hundreds of bacterial species from several sources, including soil, water and plant tissues. Until now we have succeeded in identifying two new bacterial species and one new bacterial strain [6]; Oceanobacillus jordanicus [21], Pseudomonas jordanii [22] and Bacillus paralicheniformis Strain GSFE7 [23], respectively (figure 1).
Figure 1. Whole-genome sequence-based phylogenetic tree, species cluster have the same colour code, the GC% is indicated in shades of blue, genome size and protein count is presented in black and brown bars respectively. Left: Oceanobacillus jordanicus, right: Pseudomonas jordanii.
These endophytes were analysed to determine their plant growth promoting effect (PGBE) on wheat under salinity stress. They were able to alleviate the effects of the salinity abiotic stress. Wheat seeds were able to germinate under high level of salt concentrations, and the roots, shoots and total mass parameters were clearly enhanced over the untreated control group (figure 2).
Figure 2. Plant growth promoting effect of P.jordanii on the root growth of two durum wheat genotypes (Tamaroi and Line 5004) under different levels of salinity (from left to right 0, 80, 160, 200 mM NaCl).
Several projects are running now to elucidate the mechanism in which the microorganisms affect the gene expression profile in plant in response to salt and drought stress. In addition, we carry regular genome wide association studies to identify key transcription factor families in several plant phyla [24], to understand their evolutionary history and their role in plant development, flowering, vernalization, secondary metabolite production, and abiotic stress response.
Figure 3. Maximum likelihood tree of type II MADS-box proteins in C. americana, A. thaliana, S. lycopersicum, S. indicum, and A. trichopoda. The MADS-box proteins contained in the branches for each species are indicated by different colored circles: red, C. americana; green, A. thaliana; brown, S. lycopersicum; blue, S. indicum; black, A. trichopoda.
The wet lab analysis, is augmented with in silico studies to further reveal protein-protein interaction mechanism and structure function relationship at the molecular level [25].
Figure 4. Evolution of SEP3 as a network hub. (A) Yeast three-hybrid (Y3H) assays of reconstructed ancestral and extant networks. (B) Increase of network densities in Y3H compared to Y2H networks. (C) Total amount of conserved, gained and lost interactions in addition to the gain and loss rate. (D-I) Molecular dynamic simulations for the SEP3 protein K-domain linker region demonstrating the effect of mutations on flexibility and specificity of the protein binding.
Identification and Development of Antimicrobial Agents
According to World Health Organization (WHO), antimicrobial resistance (AMR) is one of the major global threats to health and food security, in fact WHO has declared that AMR is one of the top 10 global public health threats facing humanity [26]. It is occurring all around the world, compromising our ability to cure common foodborne and other infectious diseases such as pneumonia, tuberculosis, blood poisoning, and gonorrhoea. This directly put more pressure on health systems by prolonging the hospitalization time, and requiring the use of more expensive medication. The overall daily rate of only five types of antibiotic-resistant infection was reported to be 170 per 100 000 population, which is similar to the combined burden of HIV, influenza, and tuberculosis in the same year in the EU and EAA [27]. In addition, it hampers the economy and development due to death, prolonged illness and disabilities of the work force. The WHO warns that the world is heading towards a post-antibiotic era in which common infections could once again kill [28].
Antimicrobials are the sum of, antibiotics, antivirals, antifungals and antiparasitic drugs used to treat bacterial, viral, fungal and parasitic infections, respectively. Overtime, these pathogens evolve naturally and end up developing some resistance mechanism to counter the effects of antimicrobial drugs. Many antibiotics can be bought from pharmacies without prescription, for animals and human use. This resulted in accelerated rate of drug resistance, and the appearance of pan-resistant bacteria (known as “superbugs”) which might cause infections that are not treatable with existing antimicrobial medicines such as antibiotics. Thus, a significant effort needs to be made in the field of discovering and designing new antimicrobials to mitigate this global crisis.
Key results and findings:
Recently, we have successfully isolated and identified new endophytic and rhizosphere bacterial species from the Dead Sea region [6,21-23]. These new species and several new strains might hold some valuable bacteriocins and other antimicrobial agents. Currently, the antimicrobial activity of selected members of the newly discovered endophytic and rhizospheric bacteria is being analysed against representative Gram-positive and Gram-negative bacteria. In addition to their antifungal activity against plant pathogens. Antimicrobial agents will be isolated, purified and identified using biochemical protein essays and their effective dose will be estimated. Selected bacteriocins will be synthesized at the lab (figure 5), then modelled using molecular dynamics simulation to further shed light on their structure, and possibly their mode of action at the molecular level.
Significance:
Newly discovered bacterial and fungal species might open the door for the discovery of new metabolites and antimicrobial agents that can be utilized in the field of biotechnology, pharmaceuticals and agriculture. In addition to antimicrobial effects, these newly discovered bacterial strains might hold a number of novel chemical agents such as dyes, fertilizers, anti-inflammatory and anti-cancer drugs that can be further developed into commercial products.
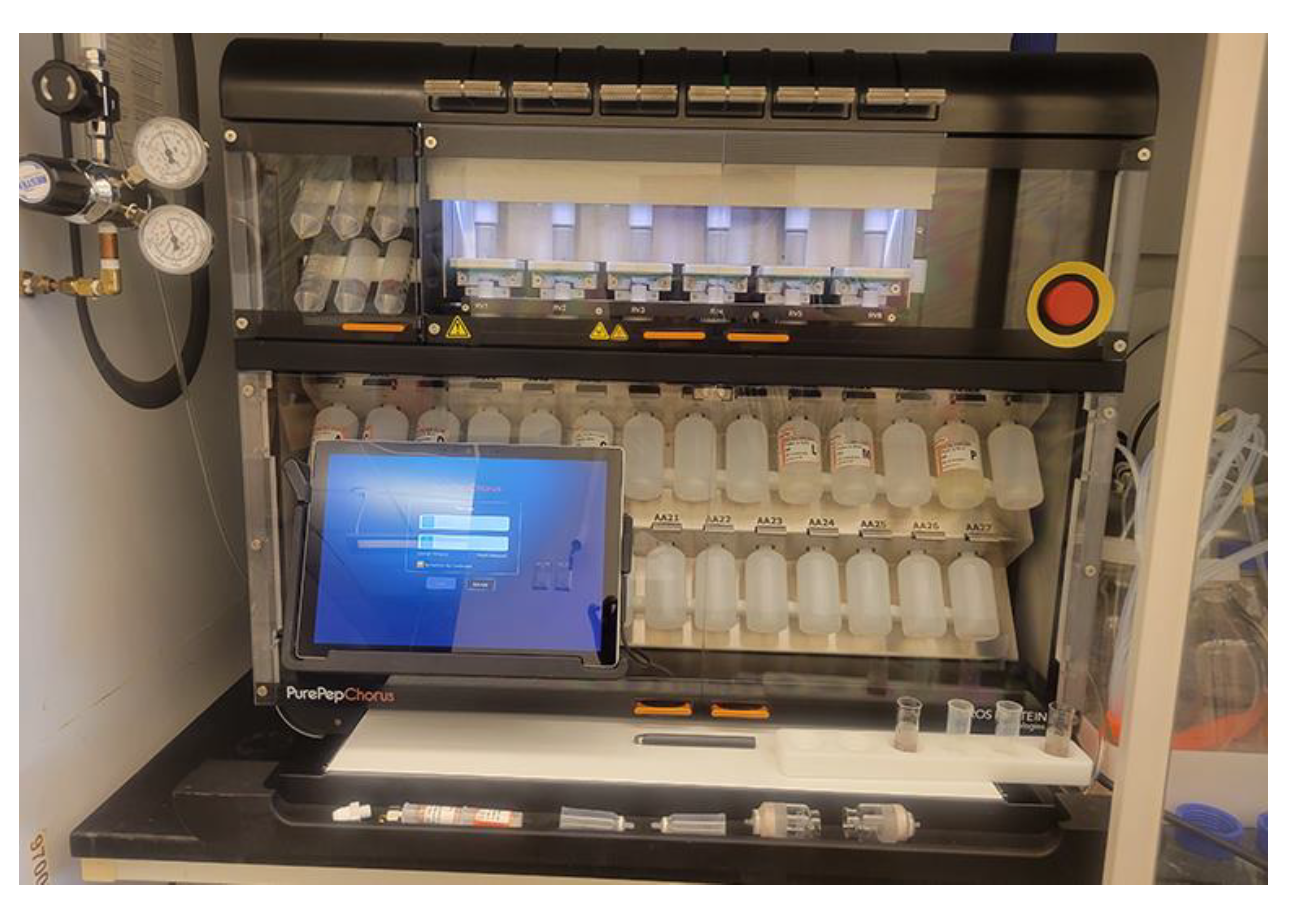
Figure 5. State of the art peptide synthesiser placed at the laboratory of Hamdi Mango Research Center. Several natural and modified oligo peptides could be easily synthesised within few days with reasonable purity and quantity.
Contact information:
Dr. Tareq Alhindi
Department of Biological Sciences
The University of Jordan
Vice Director for Hamdi Mango Centre for Scientific Research
Amman, Jordan
References:
[1] FAO, IFAD, UNICEF, WFP and WHO. 2018. The State of Food Security and Nutrition in the World 2018. Building climate resilience for food security and nutrition. Rome, FAO.
[2] FAO, IFAD, UNICEF, WFP and WHO. 2023. The State of Food Security and Nutrition in the World 2023. Urbanization, agrifood systems transformation and healthy diets across the rural–urban continuum. Rome, FAO.
[3] Zörb C, Geilfus CM, Dietz KJ. Salinity and crop yield. Plant biology. 2019 Jan;21:31-8. DOI: 10.1111/plb.12884
[4] Shrivastava P, Kumar R. Soil salinity: A serious environmental issue and plant growth promoting bacteria as one of the tools for its alleviation. Saudi journal of biological sciences. 2015;22(2):123-31. DOI: 10.1016/j.sjbs.2014.12.001
[5] Shannon MC, Grieve CM. Tolerance of vegetable crops to salinity. Scientia horticulturae. 1998 Nov 30;78(1-4):5-38.
[6] Albdaiwi RN, Khyami-Horani H, Ayad JY, Alananbeh KM, Al-Sayaydeh R. Isolation and characterization of halotolerant plant growth promoting rhizobacteria from durum wheat (Triticum turgidum subsp. durum) cultivated in saline areas of the dead sea region. Frontiers in Microbiology. 2019 Jul 23;10:1639. DOI: 10.3389/fmicb.2019.01639
[7] Grieve CM, Grattan SR, Maas EV. Plant salt tolerance. ASCE manual and reports on engineering practice. 2012;71:405-59. DOI: doi.org/10.1061/9780784411698.ch13
[8] Maas EV, Donovan TJ, Francois LE. Salt tolerance of irrigated guayule. Irrigation science. 1988 Aug;9(3):199-211. DOI: 10.1007/BF00275432
[9] Jamil A, Riaz S, Ashraf M, Foolad MR. Gene expression profiling of plants under salt stress. Critical Reviews in Plant Sciences. 2011 Sep 1;30(5):435-58. DOI: 10.1080/07352689.2011.605739
[10] Munns R, James RA, Läuchli A. Approaches to increasing the salt tolerance of wheat and other cereals. Journal of experimental botany. 2006 Mar 1;57(5):1025-43. DOI: 10.1093/jxb/erj100
[11] Etesami H, Beattie GA. Mining halophytes for plant growth-promoting halotolerant bacteria to enhance the salinity tolerance of non-halophytic crops. Frontiers in microbiology. 2018 Feb 8;9:148. DOI: 10.3389/fmicb.2018.00148
[12] Waqas M, Khan AL, Kamran M, Hamayun M, Kang SM, Kim YH, Lee IJ. Endophytic fungi produce gibberellins and indoleacetic acid and promotes host-plant growth during stress. Molecules. 2012 Sep 7;17(9):10754-73. DOI: 10.3390/molecules170910754
[13] Gamalero E, Favale N, Bona E, Novello G, Cesaro P, Massa N, Glick BR, Orozco-Mosqueda MD, Berta G, Lingua G. Screening of bacterial endophytes able to promote plant growth and increase salinity tolerance. Applied Sciences. 2020 Aug 20;10(17):5767. DOI: 10.3390/app10175767
[14] Etesami H, Glick BR. Halotolerant plant growth–promoting bacteria: Prospects for alleviating salinity stress in plants. Environmental and Experimental Botany. 2020 Oct 1;178:104124. DOI: 10.1016/j.envexpbot.2020.104124
[15] Choudhury AR, Choi J, Walitang DI, Trivedi P, Lee Y, Sa T. ACC deaminase and indole acetic acid producing endophytic bacterial co-inoculation improves physiological traits of red pepper (Capsicum annum L.) under salt stress. Journal of Plant Physiology. 2021 Dec 1;267:153544. DOI: 10.1016/j.jplph.2021.153544
[16] Ali S, Charles TC, Glick BR. Amelioration of high salinity stress damage by plant growth-promoting bacterial endophytes that contain ACC deaminase. Plant Physiology and Biochemistry. 2014 Jul 1;80:160-7. DOI: 10.1016/j.plaphy.2014.04.003
[17] Glick BR. Bacteria with ACC deaminase can promote plant growth and help to feed the world. Microbiological research. 2014 Jan 20;169(1):30-9. DOI: 10.1016/j.micres.2013.09.009
[18] Hadj Brahim A, Ben Ali M, Daoud L, Jlidi M, Akremi I, Hmani H, Feto NA, Ben Ali M. Biopriming of durum wheat seeds with endophytic diazotrophic bacteria enhances tolerance to Fusarium head blight and salinity. Microorganisms. 2022 May 5;10(5):970. DOI: 10.3390/microorganisms10050970
[19] Taheri AE, Hamel C, Gan Y. Cropping practices impact fungal endophytes and pathogens in durum wheat roots. Applied Soil Ecology. 2016 Apr 1;100:104-11. DOI: 10.1016/j.apsoil.2015.12.007
[20] Shah D, Khan MS, Aziz S, Ali H, Pecoraro L. Molecular and biochemical characterization, antimicrobial activity, stress tolerance, and plant growth-promoting effect of endophytic bacteria isolated from wheat varieties. Microorganisms. 2021 Dec 23;10(1):21. DOI: 10.3390/microorganisms10010021
[21] Alhindi T, Albdaiwi R. Draft genome sequence of Oceanobacillus jordanicus strain GSFE11, a halotolerant plant growth-promoting bacterial endophyte isolated from the Jordan valley. Evolutionary Bioinformatics. 2022 Jan;18:11769343211071114. DOI: 10.1177/11769343211071114
[22] Albdaiwi R, Alhindi T, Al-Abdallat A, Hasan S. Pseudomonas jordanii a Novel Plant Growth-Promoting Bacterial Endophyte Enhance Growth Under Salinity Stress. (in preparation)
[23] Albdaiwi R, Alhindi T, Hasan S. Draft Genome Sequence of Bacillus paralicheniformis Strain GSFE7, a Halotolerant Plant Growth-Promoting Bacterial Endophyte Isolated from Cultivated Saline Areas of the Dead Sea Region. Microbiology Resource Announcements. 2022 Sep 15;11(9):e00425-22. DOI: 10.1128/mra.00425-22
[24] Alhindi T, Al-Abdallat AM. Genome-wide identification and analysis of the MADS-box gene family in American beautyberry (Callicarpa americana). Plants. 2021 Aug 30;10(9):1805. DOI: 10.3390/plants10091805
[25] Alhindi T, Zhang Z, Ruelens P, Coenen H, Degroote H, Iraci N, Geuten K. Protein interaction evolution from promiscuity to specificity with reduced flexibility in an increasingly complex network. Scientific reports. 2017 Mar 24;7(1):44948. DOI: 10.1038/srep44948
[26] https://www.who.int/news-room/fact-sheets/detail/antimicrobial-resistance
[27] Tacconelli E, Pezzani MD. Public health burden of antimicrobial resistance in Europe. The Lancet Infectious Diseases. 2019 Jan 1;19(1):4-6. DOI: 10.1016/S1473-3099(18)30648-0
[28] https://ahpsr.who.int/publications/i/item/global-action-plan-on-antimicrobial-resistance